Hitachi Energy – Grids on the radar for accelerating US energy transition
Doug Arent, Executive Director of Strategic Public Private Partnerships at the US Department of Energy’s National Renewable Energy Laboratory and Harmeet Bawa, Hitachi Energy’s Global Head of Government and Institutional Relations share perspectives on how power systems around the world, and particularly in the US are transforming to accelerate energy transitions.
By 2035, the Biden administration announced a goal of achieving a carbon pollution-free electricity sector
Doug Arent, Executive Director of Strategic Public Private Partnerships at the US Department of Energy’s National Renewable Energy Laboratory and Harmeet Bawa, Hitachi Energy’s Global Head of Government and Institutional Relations
Global trends across the energy sector point to more clean power in the energy mix, combined with increasing electrification of end uses such as transport, heating in buildings and industrial processes. Power grids play a critical role in bringing clean power from where it is produced to where it is consumed.
The US Power Grid is vast and fairly decentralized. Like many grids around the world, it was built to connect coal and gas power plants to consumption points. Now, as renewable energy sources in the form of wind and solar are increasingly being developed across the United States, the power grid needs to evolve and modernize. With generator interconnection queues around the country growing 40 percent between 2021 and 2022, and over 1,350 GW of generation, mostly solar and wind, waiting to connect to the power grid, this evolution must accelerate.
Drawing attention to the urgency, in late 2021, the Biden administration announced a goal of achieving a carbon pollution-free electricity sector by 2035. According to some experts, achieving this goal would mean that US transmission capacity would need to more than double in just over a decade.
As the energy transition accelerates and renewable energy generation increases significantly, it is clear that we need to ensure grid capacity and flexibility to evacuate and transmit the power efficiently and reliably to consumers. We must pay urgent attention to grid infrastructure, a historically under-invested sector and realization of the message ‘no transition without transmission’ must sink in.
Looking around the world, and particularly at major economies, what has been happening recently from a policy perspective, when it comes to clean energy and decarbonization?
Harmeet Bawa: A plethora of policies which have been announced in the past few years focusing on clean energy and decarbonization highlight that governments now see energy transition as a top priority, especially if they are to achieve their ambitious Net Zero goals. Here are a few examples:
European Union:
Under the European Green Deal, the EU envisions Europe to evolve into the first climate neutral continent by 2050. To get there, the EU has developed a number of policies, directives and regulations including Fit for 55, RePower EU and the more recent Net Zero Industry Act. The focus is fully on the urgent shift towards clean power and electrification; power grids to support that shift; and the supply chains to ensure that the turbines, transformers, high-voltage direct current (HVDC) systems and other clean energy technologies needed are available.
United Kingdom:
The UK was the first G20 country to put a net zero by 2050 commitment into law. The current target for net zero power is 2035 with a 50GW of offshore wind ambition for 2030 and an end to new internal combustion engine (ICE) vehicle sales in 2030. On strategic network planning the Holistic Network Design (HND) defines the new transmission connections needed for 2030 and the Accelerated Strategic Transmission Investment (ASTI) program fast tracked their approval. The government response to Winser report is due ‘soon’ and will form an action plan to half the time taken to deliver new network infrastructure – planning, consenting, supply chains, procurement models and public communications are all expected to be an integral part of the plan.
Japan:
Japan’s Green Transformation (GX) policy aims to deliver 36-38 percent renewable energy in the country’s power mix by 2030 including 10 GW of offshore wind power and up to 118 GW of solar power. The policy also includes a plan to secure investment to maintain, up-grade and modernize transmission and distribution networks for this massive introduction of renewables. Power storage facilities, such as storage batteries (including EVs) and pumped storage, also play an important role in this policy.
Australia:
The recent energy policy, Rewiring the Nation, includes the creation of a special corporation to funnel $20bn into new transmission links to accelerate the uptake of more clean energy. The policy is designed to cut Australia’s 2005-level greenhouse gas emissions 43 percent by 2030 and achieve carbon neutrality by 2050.
What major policy announcements impacting the power sector have we seen from the US Government in recent years?
Doug Arent: Two major pieces of legislation were announced by the US government during 2021 and 2022, the Infrastructure Investment and Jobs Act (also known as the Bipartisan Infrastructure Law) and the Inflation Reduction Act.
The Infrastructure Investment and Jobs Act (IIJA) was signed into law in late 2021. The IIJA provides over $27 billion in financing and incentive programmes to improve grid resilience and reliability across the US. The aim is to build new, resilient transmission lines to facilitate the integration of renewables. The Building a Better Grid Initiative launched in Jan 2022 is a notable result of the IIJA and allocates $20 billion to deliver on this aim.
The Inflation Reduction Act (IRA) was signed into law in 2022 and contains $500 billion in new spending and tax breaks that aim to boost clean energy, reduce healthcare costs, and increase tax revenues. The IRA is heralded as the most significant climate legislation in U.S. history, accelerating the transition to a clean energy economy through a series of incentives.
According to the American Clean Power Association (ACP), initiatives in the US over the past 12 months have led to the announcement of approximately $271 billion in clean energy projects and manufacturing facilities, greater than combined clean energy investments made over the previous eight years. The implementation of these policies will be critical to reaching the US goal of 100 percent clean electricity by 2035 and a zero-emissions economy by 2050.
Concretely, how will policies such as the IIJA or the IRA impact the optimized development, refurbishment, and modernization of US transmission grids?
D.A: Both the IIJA and IRA bills set aside significant funding and authorize the US Department of Energy (DOE) to invest in modernizing the grid. Multiple offices of DOE are focused on deploying their resources to support these goals.
Additionally, DOE restructured their offices to create an Under Secretary for Infrastructure, under which are the Office of Clean Energy Demonstrations, the Grid Deployment Office, the Office of Manufacturing and Energy Supply Chains, the Office of Cybersecurity, Energy Security and Emergency Response, the Loan Programs Office and others which are all very focused on the achievement of the 2035 goals.
There is also ongoing work studying the important role, combined with the trade-offs, of enhanced transmission grids in United States as authorized in the Fiscal Responsibility Act. These would build off the Interconnections Seam Study that NREL released a number of years ago which identified positive benefit/cost ratios for a DC macro grid for the Continental United States, as well as the National Transmission Study that is currently underway.
What are two key enablers and catalysts that can accelerate the achievement of global net zero targets?
H.B: The ambitions displayed by countries around the world in recent years through their net zero commitments are laudable. This is indeed a great opportunity but nonetheless, the scale of the challenge is immense.
As the share of electricity in the energy mix increases from 20 percent to over 50 percent (even 70-80 percent by some estimates), in the global power system of 2050, we will need around four times today’s generation capacity AND we will need to transfer three times as much electrical energy.
Harmeet Bawa, Hitachi Energy’s Global Head of Government and Institutional Relations
Two key enablers to accelerate the shift towards net zero include:
1. Power Grid Investments and new business models:
Without significant and urgent acceleration of grid investments we could jeopardize our climate goals.
Through this investment, amongst other actions, we must accelerate the integration of renewables and building of interconnectors to maximize their utilization, by deploying technologies like HVDC.
Harmeet Bawa
In addition to boosting grid capacity, we also need to enhance flexibility, reliability, and grid resilience/security e.g., through digitalization across the value chain, to handle new supply and demand side complexities. Here I would like to add that when it comes to grids, a key enabler for this and the overall energy transition will be the adoption of new business models by all stakeholders including policy makers, regulators, power utilities and technology providers.
2. Deployment of technologies at Speed and Scale:
While it is important to sustain our focus on innovation & development, most technologies required for the achievement of near- and medium-term goals (e.g., to 2030 and beyond) already exist. We must focus on deployment of these technologies at ‘speed’ and ‘scale’. We need to build collaboration and coordination across stakeholders, sectors and geographies to drive the energy transition forward. We must also urgently alleviate delays relating to permitting and approvals – too many clean energy infrastructure projects have already been held up.
What are the key enablers that can accelerate achievement of the US government’s goal of 100 percent clean electricity by 2035?
D.A: There are several factors coming together, (1) technology capabilities; (2) economics, including those supported by the IRA and other recent federal legislation; and (3) market factors driving both supply and demand of clean energy, that are all building momentum toward achieving the 100 percent clean electricity by 2035 goal. NREL’s recent work, such as the 100 percent by 2035 study, or that of others such as the Princeton Net Zero study, show also that there are significant challenges in terms of scale of installation, supply chain, and building transmission, accounting for the historic timeline of doing so.
The figure below from the NREL 2035 Study highlights the significant contrast among different scenarios for transmission build out. Here we see representations of the current system side by side with either constrained build out scenarios or relatively fast transmission filled out scenarios. Transmission build-out, of course, will heavily depend upon permitting timelines, social acceptability, and other factors.
According to ACP, initiatives in the US over the past 12 months have led to the announcement of approximately $271 billion in clean energy projects and manufacturing facilities
Figure 1 – NREL 2035 Study – Transmission expansion is driven by demand growth and RE expansion even in the Reference case
DOE has also recently released a review of the US electricity grid supply chain with a focus on large power transformers and HVDC systems. The review highlighted that many critical power grid components have limited to no domestic manufacturing capacity, a challenge that policies such as the IRA are addressing.
The three major components of the U.S. power system—the Western Interconnection, the Eastern Interconnection, and the Electric Reliability Council of Texas—operate almost independently of each other. How could a nationwide high-voltage (HV) grid be developed in the US and what would be the benefits?
D.A: The Seams study, as I mentioned briefly above, evaluated multiple configurations of a HVDC macro grid for the United States. Each of these configurations had positive benefit cost ratios under a 30 percent renewable energy generation scenario. The results show benefit-to-cost ratios that reach as high as 2.5, indicating significant value to increasing the transmission capacity between the interconnections under the cases considered, realized through sharing generation resources and flexibility across regions. Additionally, the HVDC configurations offer enhanced security given the interregional transmission capabilities to address local or regional deficiencies or other balancing challenges.
For example, the study evaluated 4 configurations as depicted in the figure below. These designs reflect:
Design 1 (top left): No increase in transmission capacity between the interconnections
Design 2a (top right): Increasing capacity at existing back-to-back ties
Design 2b (bottom left): Increasing capacity at existing back-to-back ties plus three long-distance HVDC ties between the interconnections
Design 3 (bottom right): Nationwide HVDC
Details of the model can be found in this IEEE Transactions on Power Systems article.
Figure 2 – NREL’s Interconnections Seam Study – Transmission design in the capacity expansion work
The US recently announced a national goal to deploy 30 GW of offshore wind energy by 2030. What actions will be essential for the US to achieve this goal and what coordinated transmission solutions are being considered?
D.A: The offshore wind goals are envisioned to be enabled by a combination of both fixed and floating foundations, and of course efficient transmission and interconnection to key onshore locations.
The technology options for near-term deployments are quite well understood and commercial, but the permitting, interaction with ocean ecosystems and, of course, stakeholder engagement are seen as critical components of achieving these goals in a short time.
Doug Arent, Executive Director of Strategic Public Private Partnerships at the US Department of Energy’s National Renewable Energy Laboratory
Transmission configurations are under discussion and include direct connectivity, meshed grids, and hybrid configurations. Multiple efforts are launching detailed transmission planning studies. In particular, NREL is leading an effort called the Atlantic Offshore Wind Transmission Study. This study will:
- Evaluate coordinated transmission solutions to enable offshore wind deployment along the U.S. Atlantic Coast, addressing gaps in previous analyses;
- Compare different transmission technologies and topologies, quantify costs, assess reliability and resilience, and evaluate key environmental and ocean co-use issues;
- Produce timely results to inform decision making and offer feasible solutions, data, and models that may benefit stakeholders in their own planning processes.
Prior Examples include Tufts University’s Offshore Wind Transmission and Grid Interconnection across U.S. Northeast Markets – OSPRE-2021-01
What is needed to build strong and diverse cleantech grid supply chains globally?
H.B: A key enabler for building of long-gestation infrastructure like power grids will be to manage supply chains. Here we need to provide visibility and incentivize manufacturers to strengthen their footprint and boost capacities while continuing to leverage their global footprint. It has been estimated that global production capacity of clean energy technologies needs to triple for the ambitious expansion plans worldwide not to be thwarted by a shortage in key components such as wind turbines, cables, transformers, switchgear and HVDC equipment. Some supply chain related challenges such as time delays, cost increases, scarcity and high concentration of many critical minerals and key components must be overcome to enable the timely build out of energy infrastructure.
There are several actions we can take today including developing holistic forward looking and integrated approaches for long gestation infrastructure like grids, moving away from single project approvals, standardization for speeding things up and optimizing costs. We also need new business models where companies can optimize engineering resources through standardized solutions and also focus on their core competences, optimize resource usage. As a concrete example, HVDC technology providers focus on their scope and specialized firms provide the civil works and other infrastructure such as EPC services and offshore platforms in the case of Offshore wind projects. And finally, maintaining a healthy skills supply chain will be a key success factor.
How can supply chains evolve to support the US goal of 100 percent clean electricity by 2035?
D.A: The federal bills passed recently have a significant amount of support for the development of US supply chains and industry has responded quite rapidly.
More than $100 billion of investments have already been announced across solar, wind and battery storage facilities.
Doug Arent
For offshore wind, for example, announcements have been made for more than a dozen facilities and ports, and investments in a new fleet of vessels which are targeted to support the offshore wind industry.
DOE tracks announced investments at Investments in American-Made Energy | Department of Energy.
In conclusion, when it comes to Net Zero, every country has its own starting point and will follow different pathways to the common destination based on their specific circumstances. It is clear that clean electrification will drive these journeys calling for the deployment of technologies at speed and scale. As a key enabler, we must ensure timely build out of power grid infrastructure to integrate renewables, interconnect systems and manage demand-supply complexities through digital solutions. At the same time, we must ensure a ‘just’ and ‘equitable’ energy transition – delivering value to society, environment and the economy – with a shared Purpose of advancing a sustainable energy future for all.
SourceHitachi Energy
EMR Analysis
More information on Hitachi Energy: See the full profile on EMR Executive Services
More information on Claudio Facchin (Chief Executive Officer, Hitachi Energy): See the full profile on EMR Executive Services
More information on Harmeet Bawa (Global Head of Government and Institutional Relations, Hitachi Energy): See the full profile on EMR Executive Services
More information on US Department of Energy (DOE): https://www.energy.gov/ + The Department of Energy has one of the richest and most diverse histories in the Federal Government. Although only in existence since 1977, the Department traces its lineage to the Manhattan Project effort to develop the atomic bomb during World War II, and to the various energy-related programs that previously had been dispersed throughout various Federal agencies.
More information on US National Renewable Energy Laboratory (NREL) of the US Department of Energy: https://www.nrel.gov + At the National Renewable Energy Laboratory (NREL), we focus on creative answers to today’s energy challenges.
From breakthroughs in fundamental science to new clean technologies to integrated energy systems that power our lives, NREL researchers are transforming the way the nation and the world use energy.
NREL is a national laboratory of the U.S. Department of Energy.
More information on Doug Arent (Executive Director, Strategic Public Private Partnerships, National Renewable Energy Laboratory, US Department of Energy): https://www.nrel.gov/about/doug-arent.html + https://www.linkedin.com/in/doug-arent-27572/
More information on IEA (International Energy Agency): https://www.iea.org + The IEA is at the heart of global dialogue on energy, providing authoritative analysis, data, policy recommendations, and real-world solutions to help countries provide secure and sustainable energy for all.
The IEA was created in 1974 to help co-ordinate a collective response to major disruptions in the supply of oil. While oil security this remains a key aspect of our work, the IEA has evolved and expanded significantly since its foundation.
Taking an all-fuels, all-technology approach, the IEA recommends policies that enhance the reliability, affordability and sustainability of energy. It examines the full spectrum issues including renewables, oil, gas and coal supply and demand, energy efficiency, clean energy technologies, electricity systems and markets, access to energy, demand-side management, and much more.
Since 2015, the IEA has opened its doors to major emerging countries to expand its global impact, and deepen cooperation in energy security, data and statistics, energy policy analysis, energy efficiency, and the growing use of clean energy technologies.
More information on Net Zero: https://www.iea.org/reports/net-zero-by-2050 + The number of countries announcing pledges to achieve net zero emissions over the coming decades continues to grow. But the pledges by governments to date – even if fully achieved – fall well short of what is required to bring global energy-related carbon dioxide emissions to net zero by 2050 and give the world an even chance of limiting the global temperature rise to 1.5 °C. This special report is the world’s first comprehensive study of how to transition to a net zero energy system by 2050 while ensuring stable and affordable energy supplies, providing universal energy access, and enabling robust economic growth. It sets out a cost-effective and economically productive pathway, resulting in a clean, dynamic and resilient energy economy dominated by renewables like solar and wind instead of fossil fuels. The report also examines key uncertainties, such as the roles of bioenergy, carbon capture and behavioral changes in reaching net zero.
More information on Dr. Fatih Birol (Executive Director, International Energy Agency): https://www.iea.org/contributors/dr-fatih-birol
More information on The European Union: https://european-union.europa.eu/index_en + The European Union’s institutional set-up is unique and its decision-making system is constantly evolving. The 7 European institutions, 7 EU bodies and over 30 decentralised agencies are spread across the EU. They work together to address the common interests of the EU and European people.
In terms of administration, there are a further 20 EU agencies and organisations which carry out specific legal functions and 4 interinstitutional services which support the institutions.
All of these establishments have specific roles – from developing EU laws and policy-making to implementing policies and working on specialist areas, such as health, medicine, transport and the environment.
There are 4 main decision-making institutions which lead the EU’s administration. These institutions collectively provide the EU with policy direction and play different roles in the law-making process:
- the European Parliament (Brussels/Strasbourg/Luxembourg)
- the European Council (Brussels)
- the Council of the European Union (Brussels/Luxembourg)
- the European Commission (Brussels/Luxembourg/Representations across the EU)
Their work is complemented by other institutions and bodies, which include:
- the Court of Justice of the European Union (Luxembourg)
- the European Central Bank (Frankfurt)
- the European Court of Auditors (Luxembourg)
The EU institutions and bodies cooperate extensively with the network of EU agencies and organisations across the European Union. The primary function of these bodies and agencies is to translate policies into realities on the ground.
Around 60,000 EU civil servants and other staff serve the 450 million Europeans (and countless others around the world).
Currently, 27 countries are part of the EU: https://european-union.europa.eu/principles-countries-history/country-profiles_en
More information on The European Commission: https://ec.europa.eu/info/index_en + The Commission helps to shape the EU’s overall strategy, proposes new EU laws and policies, monitors their implementation and manages the EU budget. It also plays a significant role in supporting international development and delivering aid.
The Commission is steered by a group of 27 Commissioners, known as ‘the college’. Together they take decisions on the Commission’s political and strategic direction.
A new college of Commissioners is appointed every 5 years.
The Commission is organised into policy departments, known as Directorates-General (DGs), which are responsible for different policy areas. DGs develop, implement and manage EU policy, law, and funding programmes. In addition, service departments deal with particular administrative issues. Executive agencies manage programmes set up by the Commission.
Principal roles in law: The Commission proposes and implements laws which are in keeping with the objectives of the EU treaties. It encourages input from business and citizens in the law-making process and ensures laws are correctly implemented, evaluated and updated when needed.
The European Commission is the EU’s politically independent executive arm. It is alone responsible for drawing up proposals for new European legislation, and it implements the decisions of the European Parliament and the Council of the EU.
More information on Ursula von der Leyen (President, The European Commission): https://ec.europa.eu/commission/commissioners/2019-2024/president_en + https://www.linkedin.com/in/ursula-von-der-leyen/
More information on the EU Green Deal: https://ec.europa.eu/info/strategy/priorities-2019-2024/european-green-deal_en +
- The European Green Deal will transform the EU into a modern, resource-efficient and competitive economy, ensuring:
- no net emissions of greenhouse gases by 2050
- economic growth decoupled from resource use
- no person and no place left behind
- The European Green Deal provides an action plan to:
- boost the efficient use of resources by moving to a clean, circular economy
- restore biodiversity and cut pollution
- The plan outlines investments needed and financing tools available. It explains how to ensure a just and inclusive transition.
- The EU aims to be climate neutral in 2050. We proposed a EuropeanClimate Law to turn this political commitment into a legal obligation.
More information on the EU Fit-for-55 package: The Fit for 55 Package encompasses a suite of legislative initiatives across various sectors, including energy, transport and buildings, which is intended to fundamentally overhaul the EU’s climate policy framework and put the EU on track to deliver on its 2030 climate target of 55%.
More information on RePower EU: https://commission.europa.eu/strategy-and-policy/priorities-2019-2024/european-green-deal/repowereu-affordable-secure-and-sustainable-energy-europe_en + Affordable, secure and sustainable energy for Europe.
In response to the hardships and global energy market disruption caused by Russia’s invasion of Ukraine, the European Commission is implementing its REPowerEU Plan.
Launched in May 2022, REPowerEU is helping the EU
- save energy
- produce clean energy
- diversify its energy supplies
Thanks to REPowerEU, we’ve safeguarded EU citizens and businesses from energy shortages, supported Ukraine by weakening Russia’s war chest, and accelerated the transition to clean energy. Our joint efforts continue and Europe is now better prepared and more united than ever.
Putin’s attempt to blackmail Europe using energy has failed.
More information on the Net-Zero Industrial Act (NZIA): https://single-market-economy.ec.europa.eu/publications/net-zero-industry-act_en + Proposal for a regulation of the European Parliament and of the Council on establishing a framework of measures for strengthening Europe’s net-zero technology products manufacturing ecosystem (Net Zero Industry Act)
More information on the UK Accelerated Strategic Transmission Investment (ASTI) Program: https://www.ofgem.gov.uk/publications/decision-accelerating-onshore-electricity-transmission-investment + The British Energy Security Strategy set out the Government’s ambition to connect up to 50GW of offshore generation to the electricity network by 2030. Facilitating this ambition will require significant reinforcements to the onshore electricity transmission network and a change to the current regulatory framework in order to accelerate delivery of large projects.
In August 2022 we consulted on how Ofgem could support the accelerated delivery of the strategic electricity transmission network upgrades needed to meet the Government’s 2030 renewable electricity generation ambitions.
This document contains our decision to introduce a new Accelerated Strategic Transmission Investment (ASTI) framework. We set out the initial list of ASTI projects, our decision on exempting strategic projects from competition, the new process for assessing and funding ASTI projects and the range of measures we are introducing to protect consumers against additional risks that changing the process brings.
More information on Japan’s Green Transformation (GX) Initiative: https://grjapan.com/sites/default/files/content/articles/files/gr_japan_overview_of_gx_plans_january_2023.pdf + Green Transformation, better known as “GX,” is an initiative led by the Japanese government to supposedly bring about a shift from an industrial structure centered on fossil energy to one centered on clean energy.
On 10 February 2023, the Japanese Cabinet confirmed the Basic Plan for the GX: Green Transformation Policy, aimed to help Japan achieve its climate goals.
More information on Joe Biden (46th President, USA): https://www.whitehouse.gov/administration/president-biden/
More information on The US Infrastructure Investment and Jobs Act (IIJA): https://transportation.house.gov/committee-activity/issue/infrastructure-investment-and-jobs-act#:~:text=The%20Infrastructure%20Investment%20and%20Jobs,create%20good%2Dpaying%20union%20jobs. + The Infrastructure Investment and Jobs Act means historic investment that will modernize our roads, bridges, transit, rail, ports, airports, broadband, and drinking water and wastewater infrastructure.
This legislation does not raise taxes on everyday Americans, and it will create good-paying union jobs. In fact, investments made in the Infrastructure Investment and Jobs Act, combined with those in the Build Back Better Act, will add an average of 1.5 million jobs each year for the next decade, renewing America’s promise of shared prosperity.
The investments in this legislation will also help create more livable communities by reducing carbon pollution from the transportation sector and helping to improve water and air quality. The bill provides $550 billion in NEW spending on our nation’s infrastructure over the next five years.
More information on The US Bipartisan Infrastructure Law: https://www.whitehouse.gov/bipartisan-infrastructure-law/ + Congress passed the Bipartisan Infrastructure Law (Infrastructure Investment and Jobs Act), a once-in-a-generation investment in our nation’s infrastructure and competitiveness.
This Bipartisan Infrastructure Law will rebuild America’s roads, bridges and rails, expand access to clean drinking water, ensure every American has access to high-speed internet, tackle the climate crisis, advance environmental justice, and invest in communities that have too often been left behind. The legislation will help ease inflationary pressures and strengthen supply chains by making long overdue improvements for our nation’s ports, airports, rail, and roads. It will drive the creation of good-paying union jobs and grow the economy sustainably and equitably so that everyone gets ahead for decades to come. Combined with the President’s Build Back Framework, it will add on average 1.5 million jobs per year for the next 10 years.
This historic legislation will:
- Deliver clean water to all American families and eliminate the nation’s lead service lines
- Ensure every American has access to reliable high-speed internet.
- Repair and rebuild our roads and bridges with a focus on climate change mitigation, resilience, equity, and safety for all users.
- Improve transportation options for millions of Americans and reduce greenhouse emissions through the largest investment in public transit in U.S. history.
- Upgrade our nation’s airports and ports to strengthen our supply chains and prevent disruptions that have caused inflation.
- Make the largest investment in passenger rail since the creation of Amtrak.
- Build a national network of electric vehicle (EV) chargers.
- Upgrade our power infrastructure to deliver clean, reliable energy across the country and deploy cutting-edge energy technology to achieve a zero-emissions future.
- Make our infrastructure resilient against the impacts of climate change, cyber-attacks, and extreme weather events.
- Deliver the largest investment in tackling legacy pollution in American history by cleaning up Superfund and brownfield sites, reclaiming abandoned mines, and capping orphaned oil and gas wells.
More information on the U.S. Inflation Reduction Act (IRA): https://www.epa.gov/green-power-markets/inflation-reduction-act + https://www.wri.org/update/brief-summary-climate-and-energy-provisions-inflation-reduction-act-2022 + The Inflation Reduction Act of 2022 (IRA) is the most significant climate legislation in U.S. history. IRA’s provisions will finance green power, lower costs through tax credits, reduce emissions, and advance environmental justice.
The Inflation Reduction Act (IRA), which was signed into law in August 2022, will cut Americans’ energy costs, create good jobs and transform U.S. efforts to address the climate crisis. It is the largest single step that Congress has ever taken to address climate change. The IRA includes nearly $370 billion in investments in disadvantaged communities, prioritizing projects that repurpose retired fossil fuel infrastructure and employ displaced workers, setting the U.S. on a course toward a fair, equitable and economic clean energy transition.
More information on the U.S. Fiscal Responsibility Act: https://www.congress.gov/bill/118th-congress/house-bill/3746/summary/00 + This bill increases the federal debt limit, establishes new discretionary spending limits, rescinds unobligated funds, and expands work requirements for federal programs.
Specifically, the bill suspends the federal debt limit through January 1, 2025, and increases the limit on January 2, 2025, to accommodate the obligations issued during the suspension period.
In addition, the bill establishes new discretionary spending limits for FY2024 and FY2025 that are enforced with sequestration (i.e., automatic spending cuts). It also changes the limits to 1% below the FY2023 base funding levels if a continuing resolution is in effect on or after January 1, 2024, or on or after January 1, 2025, because all 12 regular appropriations bills were not enacted by the end of the prior year.
More information on The American Clean Power Association (ACP): https://cleanpower.org/ + American Clean Power is the voice of companies from across the clean power sector that are powering America’s future and providing cost-effective solutions to the climate crisis while creating jobs, spurring massive investment in the U.S. economy, and driving high-tech innovation across the nation.
More information on Jason Grumet (Chief Executive Officer, The American Clean Power Association (ACP)): https://cleanpower.org/about/team/jason-grumet/ + https://www.linkedin.com/in/grumet/
More information on the US National Transmission Planning Study from the US Department of Energy (DOE): https://www.energy.gov/gdo/national-transmission-planning-study + In support of the Infrastructure Investment and Jobs Act, DOE’s Office of Electricity launched the Building a Better Grid Initiative to catalyze the nationwide development of new and upgraded high-capacity electric transmission lines.
As one of the first steps, DOE is conducting the National Transmission Planning Study (NTP) to identify transmission that will provide broad-scale benefits to electric customers; inform regional and interregional transmission planning processes; and identify interregional and national strategies to accelerate decarbonization while maintaining system reliability.
In partnership with the Pacific Northwest National Laboratory and the National Renewable Energy Laboratory, DOE will collaborate with industry stakeholders, communities, and regional and local governments to help identify pathways for necessary large-scale transmission system buildouts that meet regional and national interests.
EMR Additional Notes:
- Grid, Microgrids and DERs:
- The power grid is a network for delivering electricity to consumers. The power grid includes generator stations, transmission lines and towers, and individual consumer distribution lines.
- The grid constantly balances the supply and demand for the energy that powers everything from industry to household appliances.
- Electric grids perform three major functions: power generation, transmission, and distribution.
- A microgrid is a small-scale power grid that can operate independently or collaboratively with other small power grids. The practice of using microgrids is known as distributed, dispersed, decentralized, district or embedded energy production.
- Smart Grid is any electrical grid + IT at all levels . Micro Grid is a group of interconnected loads and DERs (Distributed energy resources) within a clearly defined electrical and geographical boundaries witch acts as a single controllable entity with respect to the main grid.
- Distributed energy resources (DERs) are small-scale electricity supply (typically in the range of 3 kW to 50 MW) or demand resources that are interconnected to the electric grid. They are power generation resources and are usually located close to load centers, and can be used individually or in aggregate to provide value to the grid.
- Common examples of DERs include rooftop solar PV units, natural gas turbines, microturbines, wind turbines, biomass generators, fuel cells, tri-generation units, battery storage, electric vehicles (EV) and EV chargers, and demand response applications.
- Distributed energy resources management systems (DERMS) are platforms which helps mostly distribution system operators (DSO) manage their grids that are mainly based on distributed energy resources (DER).
- DERMS are used by utilities and other energy companies to aggregate a large energy load for participation in the demand response market. DERMS can be defined in many ways, depending on the use case and underlying energy asset.
- Carbon Dioxide (CO2):
- Primary greenhouse gas emitted through human activities. Carbon dioxide enters the atmosphere through burning fossil fuels (coal, natural gas, and oil), solid waste, trees and other biological materials, and also as a result of certain chemical reactions (e.g., manufacture of cement). Carbon dioxide is removed from the atmosphere (or “sequestered”) when it is absorbed by plants as part of the biological carbon cycle.
- Decarbonization:
- Reduction of carbon dioxide emissions through the use of low carbon power sources, achieving a lower output of greenhouse gasses into the atmosphere.
- Substation:
- A power station is where the power is generated. A sub station is where power is split apart, distributed and spread further into the grid.A substation is a part of an electrical generation, transmission, and distribution system.
- Substations contain the specialist equipment that allows the voltage of electricity to be transformed (or ‘switched’). The voltage is stepped up or down through pieces of equipment called transformers, which sit within a substation’s site.
- Transformers (Distribution Transformers and Power Transformers):
- A distribution transformer is the type of transformer that performs the last voltage transformation in a distribution grid. It converts the voltage used in the transmission lines to one suitable for household and commercial use, typically down to 240 volts.
- The transformer is classified into three types based on the voltage level produced: Step down, Step up, and an isolation transformer.
- Transformers changes from high voltage to low voltage, used in homes and businesses. The main function of this is to reduce the voltage to provide isolation between the two windings as primary and secondary. This transformer distributes electricity to remote areas generated from power plants.
- While transformer stations are linked to high/medium-voltage transmission systems, electrical substations are designed to support and transform lower voltages.
- Distribution transformers always operate at a load less the rated full load. Power transformers always operate at full load. Distribution transformers are designed to give maximum efficiency at 60 to 70% of the rated load. Power transformers have maximum efficiency at full load.
- Power Transformers are used in transmission network of higher voltages for step-up and step down application (400 kV, 200 kV, 110 kV, 66 kV, 33kV) and are generally rated above 200MVA.
- Distribution Transformers are used for lower voltage distribution networks as a means to end user connectivity. (11kV, 6.6 kV, 3.3 kV, 440V, 230V) and are generally rated less than 200 MVA.
- Shunt Reactor:
- Shunt reactors (SRs) are used in high voltage energy transmission systems to control the voltage during load variations. Depending on the voltage requirement needs, shunt reactors are switched on or off to provide reactive power compensation.
- A shunt reactor is an absorber of reactive power, thus, increasing the energy efficiency of the system. It is the most compact device commonly used for reactive power compensation in long high-voltage transmission lines and in cable systems. The shunt reactor can be directly connected to the power line or to a tertiary winding of a three-winding transformer. The shunt reactor could be permanently connected or switched via a circuit breaker.
- Shunt reactor is same as power transformer but it has only one winding per phase as compared to power transformer. Shunt reactors are used to increase the power and energy system efficiency as it absorb & compensate the reactive power in cables and long high voltage transmission lines.

- HVDC Light:
- HVDC Light is the successful and environmentally-friendly way to design a power transmission system for a submarine cable, an underground cable, using over head lines or as a back-to-back transmission. HVDC Light is HVDC technology based on voltage source converters (VSCs).
- HVDC Light is designed to transmit power underground and underwater, also over long distances. It offers numerous environmental benefits, including “invisible” power lines, neutral electromagnetic fields, oil-free cables and compact converter stations.
- As its name implies, HVDC Light is a dc transmission technology. However, it is different from the classic HVDC technology used in a large number of transmission schemes. Classic HVDC technology is mostly used for large point-to-point transmissions, often over vast distances across land or under water. It requires fast communications channels between the two stations, and there must be large rotating units – generators or synchronous condensers – present in the AC networks at both ends of the transmission. HVDC Light consists of only two elements: a converter station and a pair of ground cables. The converters are voltage source converters, VSC’s. The output from the VSC’s is determined by the control system, which does not require any communications links between the different converter stations. Also, they don’t need to rely on the AC network’s ability to keep the voltage and frequency stable. These feature make it possible to connect the converters to the points bests suited for the AC system as a whole.
- HVDC (High-Voltage Direct Current):
- Key enabler for a carbon-neutral energy system. It is highly efficient for transmitting large amounts of electricity over long distances, integration of renewables and interconnecting grids, opening up for new sustainable transmission solutions.
- HVDC Links:
- The first successful HVDC experimental long distance line (37 miles) was made at Munich, Germany in 1882 by Oskar Von Miller and fellow engineers.
- HVDC allows power transmission between AC transmission systems that are not synchronized. Since the power flow through an HVDC link can be controlled independently of the phase angle between source and load, it can stabilize a network against disturbances due to rapid changes in power.
- An HVDC line has considerably lower losses compared to HVAC over longer distances.
- Neu Connect (the first power interconnection between Great Britain and Germany): https://neuconnect-interconnector.com +
- The NeuConnect Interconnector will create the first direct power link between Germany and Great Britain, connecting two of Europe’s largest energy markets for the first time. Around 720km of land and subsea cables will form an ‘invisible highway’ allowing up to 1.4GW of electricity to move in either direction, enough to power up to 1.5 million homes over the life of the project.
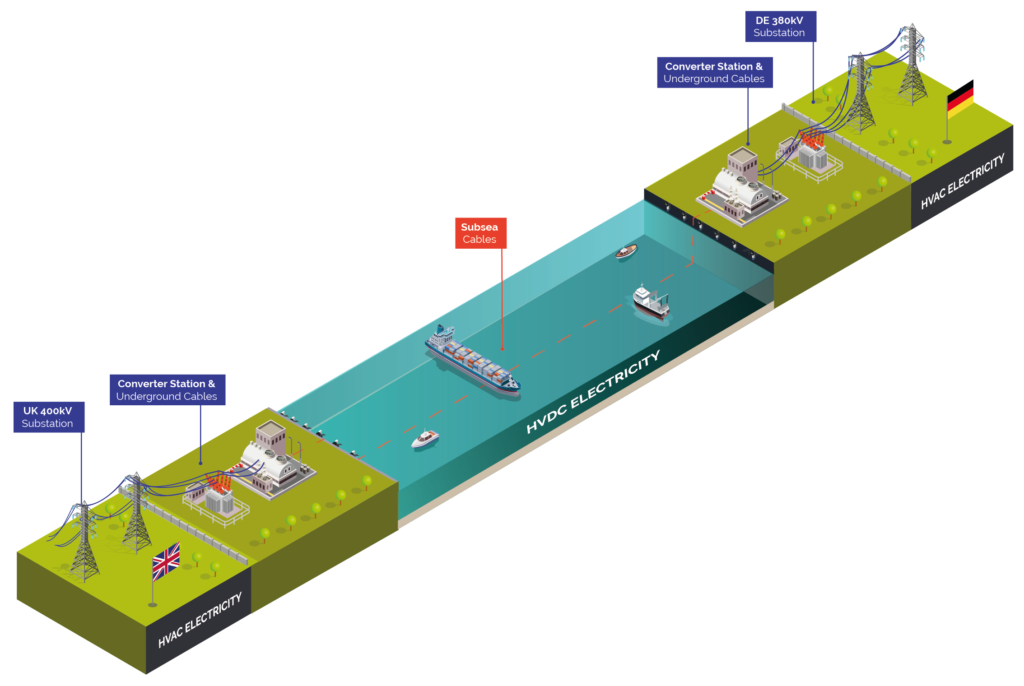
- The Tyrrhenian Link: https://www.terna.it/en/projects/public-engagement/Tyrrhenian-link +
- Connecting Sicily with Sardinia and the Italian peninsula via a double underwater cable: a new electricity corridor at the centre of the Mediterranean; the Tyrrhenian Link. At 950 kilometres long and with a capacity of 1000 MW, this is an infrastructure initiative of international significance, another step towards a more sustainable energy future. The link will improve electricity exchange capacity, facilitate the development of renewable energy sources, and the reliability of the grid.
- The overall project involves two sections: EAST from Sicily to Campania and WEST from Sicily to Sardinia.
- The East section is 480 kilometres long and connects the Fiumetorto landing point, in the municipality of Termini Imerese in Sicily, with the landing point in Torre Tuscia Magazzeno, near Battipaglia in Campania.
- The WEST section is approximately 470 kilometres long and connects the Fiumetorto landing point to the one in Terra Mala, in Sardinia.
- The Viking Link: https://viking-link.com +
- World’s longest power interconnection. the Viking Link is a 1400 MW high voltage direct current (DC) electricity link between the British and Danish transmission systems connecting at Bicker Fen substation in Lincolnshire and Revising substation in southern Jutland, Denmark.
- The North Sea Link: https://northsealink.com/ + North Sea Link is a 720 kilometre subsea interconnector linking the electricity systems of the UK and Norway. The 1400 megawatt interconnector stretches from Blyth in the UK, across the North Sea, to Kvilldal in Norway.
- The Hertel–New York interconnection line: https://www.hydroquebec.com/projects/hertel-new-york-interconnection/ + The Hertel–New York interconnection line project aims to supply clean, renewable energy to New York City. In Québec, the project involves the construction of a line that will span 57.7 km (56.1 km underground and 1.6 km underwater). This 400-kV direct current line will connect Hertel substation in La Prairie to an interconnection point in the Rivière Richelieu at the Canada–United States border.
- The Biscay Gulf Project: https://www.inelfe.eu/en/projects/bay-biscay + The electricity interconnection between Gatika (Spain) and Cubnezais (France) will be the first fundamentally submarine interconnection between Spain and France. This project will increase the exchange capacity from 2,800 to 5,000 MW, improving the safety, stability and quality of electricity supply between the two countries and also with the rest of Europe.
- The Eastern Green Link 2 (EGL2): https://www.ssen-transmission.co.uk/projects/project-map/eastern-green-link-2/ + The Eastern Green Link 2 project is a proposal to install a sub-sea high-voltage direct current (HVDC) cable from Sandford Bay, at Peterhead, to Drax in England. There is currently a large amount of forecasted generation that will require connection to the electricity network in the coming years and as such we are proposing several upgrades to the transmission network across the north of Scotland to facilitate this. The Eastern HVDC Link will play a key role in helping achieve our Net-Zero targets.

- The EuroAsia Interconnector: https://euroasia-interconnector.com/ + EuroAsia Interconnector Limited is the official EU project developer of the 2,000MW electricity interconnector between Israel, Cyprus, Greece and Europe. The EuroAsia Interconnector is a leading European Project of Common Interest (PCI) labelled as an EU “electricity highway” connecting the national electricity grids of Israel, Cyprus and Greece through a 1,208 km subsea HVDC cable.
- The EuroAsia Interconnector comprises the electricity interconnection between the grids of Israel, Cyprus, Greece through a subsea DC cable and with HVDC onshore converter stations at each connection point, with a total capacity of 2000MW. The project is an energy highway bridging Asia and Europe, with a total length of 1,208 km. It creates a reliable alternative route for the transfer of electric energy to and from Europe.

- AC (Alternating Current) & DC (Direct Current) & UC (Universal Current):
- Direct current (DC) is an electric current that is uni-directional, so the flow of charge is always in the same direction. As opposed to alternating current, the direction and amperage of direct currents do not change. It is used in many household electronics and in all devices that use batteries.
- Direct current has many uses, from the charging of batteries to large power supplies for electronic systems, motors, and more. Very large quantities of electrical energy provided via direct-current are used in smelting of aluminum and other electrochemical processes.
- in contrast to AC power, DC power is entirely made up of active power, meaning that there are almost no losses due to the capacitance of wires when DC power travels long distances. In fact, high voltage AC transmission systems have losses of 7% to 15% with aboveground transmission.
- Alternating Current is used in homes as Direct current can not be easily stepped up or stepped down with the help of transformers whereas alternating current can easily be converted from low voltage to high voltage or vice-versa with the help of transformers.
- “UC” is used for “Universal Current”, that translates to “either DC or AC”. So a 24 V UC input can accept either 24 V AC or 24 V DC.
- Low-Voltage (LV):
- The International Electrotechnical Commission (IEC) defines supply system low voltage as voltage in the range 50–1000 V AC or 120–1500 V DC.
- Medium-Voltage (MV):
- Medium-voltage circuit breakers rated between 1 and 35/72 kV.
- High-Voltage (HV):
- The International Electrotechnical Commission define high voltage as above 1000 V for alternating current, and at least 1500 V for direct current.
- Super High-Voltage:
- Is >300kV.
- Ultra High-Voltage:
- Is >1.000kV.
- HND (Holistic Network Design) :
- The Holistic Network Design (HND) is a first of its kind, integrated approach for connecting 23GW of offshore wind to Great Britain.
- By considering future offshore generation out to 2030, infrastructure can be planned to bring power to the grid cohesively, ensuring maximum benefit for consumers, local communities and the environment.
- The HND provides a recommended offshore and onshore design for a 2030 electricity network, that facilitates the Government’s ambition for 50GW of offshore wind by 2030.
- The HND enables investment and delivery of infrastructure, including locations in North and South Wales, the Scottish Islands and West Coast, and the East Coast of Scotland and Aberdeenshire, Lancashire, North-East England, and Yorkshire & Humber, opening the door for more jobs and economic growth in these regions.
- The recommended design in the HND has equally considered four different objectives to make sure the most appropriate approach is taken forwards, including:
- Cost to consumer
- Deliverability and operability
- Impact on environment
- Impact on local communities
- Kilovolt-Amperes (kV):
- Kilovolt or “kV” means a unit of potential difference equal to 1,000 volts. Kilovolt or “kV” means the potential difference between two points on a conductor carrying a current of one ampere when the power dissipated between the two points is one kilovolt-ampere. Kilovolt means one thousand volts (kV).
- Kilovolt (kVA):
- kVA stands for Kilo-volt-amperes – a term used for the rating of an electrical circuit. kVA is the product of the circuits maximum current and voltage rating. It is also known as Apparent Power.
- kW is the unit of real power and kVA is a unit of apparent power (or real power plus re-active power). The power factor, unless it is defined and known, is therefore an approximate value (typically 0.8), and the kVA value will always be higher than the value for kW.
- A kVA is 1,000 volt-amps. It’s what you get when you multiply the voltage (the force that moves electrons around a circuit) by the amps (electrical current).
- Kilowatt (kW):
- A kilowatt is simply a measure of how much power an electric appliance consumes—it’s 1,000 watts to be exact. You can quickly convert watts (W) to kilowatts (kW) by diving your wattage by 1,000: 1,000W 1,000 = 1 kW.
- Gigawatt (GW):
- A gigawatt (GW) is a unit of power, and it is equal to one billion watts.
- According to the Department of Energy, generating one GW of power takes over three million solar panels or 310 utility-scale wind turbines
- Switchgears:
- Broad term that describes a wide variety of switching devices that all fulfill a common need: controlling, protecting, and isolating power systems. This definition can be extended to include devices to regulate and meter a power system, circuit breakers, and similar technology.
- Switchgear contains fuses, switches, and other power conductors. However, circuit breakers are the most common component found in switchgear.
- Performs the function of controlling and metering the flow of electrical power in addiction to acting as interrupting and switching devices that protects the equipment from damage arising out of electrical fluctuations.
- There are three types of switch gears namely LV (Low voltage), MV (Medium voltage) and HV (High voltage) Switchgear.
- Circuit Breakers:
- Mechanical electrical switch designed to protect an electrical circuit from damage caused by overcurrent/overload or short circuit. Its basic function is to interrupt current flow after protective relays detect a fault.
- By definition a circuit breaker is an electrical safety device, a switch that automatically interrupts the current of an overloaded electric circuit, ground faults, or short circuits.
- Fuses:
- Single time mechanical circuit interruption in an over-current situation through fusion of a graded electrical conductor. Employed in 30KV to 100KV range.
- Electrical safety device that operates to provide overcurrent protection of an electrical circuit. Its essential component is a metal wire or strip that melts when too much current flows through it, thereby stopping or interrupting the current.
- ACB (Air Circuit Breakers):
- Uses air as insulating medium.
- Air circuit breaker is a circuit breaker for the purpose of protecting low voltage circuit, mainly for energizing and cutting off high current
- VCB (Vacuum Circuit Breakers):
- Vacuum is used as the means to protect circuit breakers.
- Circuit breaker where the arc quenching takes place in a vacuum medium. The operation of switching on and closing of current carrying contacts and interrelated arc interruption takes place in a vacuum chamber in the breaker which is called a vacuum interrupter.
- AIS (Air Insulated Switchgears):
- Air is used for insulation in a metal-clad system
- Secondary power distribution device and medium voltage switchgear that helps redistribute the power of a primary power distributor powered by a high voltage distribution transformer. AIS controls, protects and isolates electrical equipment in power transmission and distribution systems.
- GIS (Gas Insulated Switchgears):
- All working components assembled under SF6 (Sulfur Hexafluoride HV Switchgears) gas-tight casing.
- Compact metal encapsulated switchgear consisting of high-voltage components such as circuit-breakers and disconnectors, which can be safely operated in confined spaces.
- OCB (Oil Circuit Breakers):
- Vapors a portion of oil to blast a jet of oil through the arc.
- Circuit breaker which uses insulating oil as an arc quenching medium
- Hybrid Circuit Breakers:
- Combines Air-insulated and SF6 Gas-insulated technologies.
- MCB (Miniature Circuit Breakers):
- Employed in domestic households to safeguard against overload. Rated current max. 100 A.
- Electrical switch that automatically switches off the electrical circuit during an abnormal condition of the network means an overload condition as well as a faulty condition. Nowadays we use an MCB in a low-voltage electrical network instead of a fuse.
- Circuit breakers have a tripping relay mechanism, while MCB has a tripping release mechanism. Circuit breakers have a high rupturing capacity, but the MCB has a low rupturing capacity. Circuit breakers are used in High Voltage systems, while MCBs are used in Low Voltage systems.
- RCCB (Residual Current Circuit Breakers):
- To safeguard against electrical shock arising out of indirect contact and includes the detection of residual current such as earth leakage.
- Current sensing device, which can automatically measure and disconnect the circuit whenever a fault occurs in the connected circuit or the current exceeds the rated sensitivity.
- MCCB (Molded Case Circuit Breakers):
- Incorporates insulating material in the form of molded casing within circuit breaker. Rated current up to 2,500 A.
- MCCB has a higher interrupting capacity, meaning it can handle larger loads than a conventional breaker. Generally, a standard breaker is used for residential and light commercial applications, while an MCCB is suitable for industrial and heavy commercial applications.
- Disconnectors:
- Automatic switching device that offers specific isolating distance on the basis of specific requirements.
- Disconnectors (also known as Isolators) are devices which are generally operated off-load to provide isolation of main plant items for maintenance, or to isolate faulted equipment from other live equipment.
- Contactors:
- Works alike high-current switching systems but at higher voltage rates. Contactors can however not be utilized as disconnecting switches. Contactors are employed in 30KV to 100KV range.
- Special type of relay used for switching an electrical circuit on or off.
- Electrical device that is widely used for switching circuits on and off. As such, electrical contactors form a subcategory of electromagnetic switches known as relays. A relay is an electrically operated switching device that uses an electromagnetic coil to open and close a set of contacts.
- PTCB eFuse Circuit Breaker:
- Electronic micro fuse for DIN rail protecting electronically nominal currents below 1A to facilitate the clear detection of faults and supports precise fault localization and fast recovery. Response times are shorter compared to conventional fuse protection and the exact current value can be adjusted at any time
- RCD (Residual Current Devices):
- Sensitive safety device that switches off the electricity within 10 to 50 milliseconds if there is an electrical fault. An RCD is is designed to protect against the risks of electrocution and fire caused by earth faults.
- The difference between a circuit breaker and an RCD switch is the purpose of a circuit breaker is to protect the electrical systems and wiring in a home while the purpose of an RCD switch is to protect people from electrocution.
- RCBO (Residual Current Breaker with Over-Current):
- RCDs can protect against electric shocks, residual currents, and earth faults. On the other hand, RCBOs can do what RCDs can do and protect a circuit from short circuits and overload. RCBOs are essentially a combination of MCB and RCCB.
- An RCBO protects electrical equipment from two types of faults; residual current and over current. Residual current, or Earth leakage as it can sometimes be referred to, is when there is a break in the circuit that could be caused by faulty electrical wiring or if the wire is accidentally cut.
- Ring Main Unit (RMU):
- Medium voltage, gas-insulated, fully sealed cabinet used to measure, connect, and integrate transformer protection functions with a fixed type breaker. Ring Main Units are safe, reliable, low-maintenance, and easy to replace switchgear.
- A ring main unit (RMU) is a factory assembled, metal enclosed set of switchgear used at the load connection points of a ring-type distribution network.
- Load Center – Panel Board – Switch Board:
- A load center is used in residential and light commercial applications to distribute electricity supplied by the utility company throughout the home or building to feed all the branch circuits. Each branch circuit is protected by the circuit breaker housed in the load center. In the event of a short circuit or an overload on a branch circuit, the circuit breaker will cut the power before any potential property damage or personal injury can occur.
- A load center provides similar functionality in a power distribution system as a switchboard and a panelboard. As far as UL and the NEC standards are concerned, there is no difference between a panelboard and a load center.
- However, Panelboards are typically deeper than load centers and can accommodate both bolt-on circuit breakers as well as plug-in breakers, whereas a load center is limited to plug-in breakers.
- Switchboards are often the typical choice for industrial establishments. These panelboards generally house circuit breakers that can manage and supply electricity for machines with high-voltage demands.
- Panelboards are only accessible from the front (as mentioned above), but switchboards allow rear access as well.

- EPC (Engineering, Procurement and Construction):
- An Engineering, Procurement and Construction Contract (“EPC Contract”), occasionally known as a “Turnkey Contract”, is a construction contract where the contractor (“EPC Contractor”) holds the responsibility towards the design, procurement, construction, commissioning and handover of a project (“Project”).
- An EPC contractor performs the engineering, procurement and construction scope(s) for a project, i.e. the EPC executes the actual work.
- Engineering, Procurement, Construction and Installation (EPCI):
- Also referred to as an engineering, procurement, construction and installation contract, this is a contract under which a principal engages a contractor to design, build, deliver and install the asset in order for it to be operational.
- EPCI contracts are often used for large-scale mining infrastructure such as mineral processing plants.
- EPCI contracts are complex because they encompass the design, detailed engineering and construction of infrastructure, including the supply of materials and services (for example, testing and installation) to ensure the smooth operation of the infrastructure.
- Under an EPCI contract, the contractor may perform all the services itself; however, EPCI contracts usually give the contractor a right to subcontract part of the work.
- However, the contractor bears the project risk because most EPCI contracts are for a fixed price (irrespective of subcontractor agreements) and based on a schedule mutually agreed in the EPCI contract. The contract price paid by the principal to the contractor is usually paid in stages on the completion of certain project milestones.