IEA – World Energy Outlook 2024
Geopolitical tensions are laying bare fragilities in the global energy system, reinforcing need for faster expansion of clean energy
World Energy Outlook 2024 shows critical choices facing governments and consumers as period of more ample supplies nears and surging electricity demand reshapes energy security
Regional conflicts and geopolitical strains are highlighting significant fragilities in today’s global energy system, making clear the need for stronger policies and greater investments to accelerate and expand the transition to cleaner and more secure technologies, according to the IEA’s new World Energy Outlook 2024.
The latest edition of the World Energy Outlook (WEO), the most authoritative global source of energy analysis and projections, examines how shifting market trends, evolving geopolitical uncertainties, emerging technologies, advancing clean energy transitions and growing climate change impacts are all changing what it means to have secure energy systems. In particular, the new report underscores that today’s geopolitical tensions and fragmentation are creating major risks both for energy security and for global action on reducing greenhouse gas emissions.
The report’s projections based on today’s policy settings indicate that the world is set to enter a new energy market context in the coming years, marked by continued geopolitical hazards but also by relatively abundant supply of multiple fuels and technologies. This includes an overhang of oil and liquefied natural gas (LNG) supply coming into view during the second half of the 2020s, alongside a large surfeit of manufacturing capacity for some key clean energy technologies, notably solar PV and batteries.
“In the second half of this decade, the prospect of more ample – or even surplus – supplies of oil and natural gas, depending on how geopolitical tensions evolve, would move us into a very different energy world from the one we have experienced in recent years during the global energy crisis,” said IEA Executive Director Fatih Birol. “It implies downward pressure on prices, providing some relief for consumers that have been hit hard by price spikes. The breathing space from fuel price pressures can provide policymakers with room to focus on stepping up investments in clean energy transitions and removing inefficient fossil fuel subsidies. This means government policies and consumer choices will have huge consequences for the future of the energy sector and for tackling climate change.”
Based on today’s policy settings, the report finds that low-emissions sources are set to generate more than half of the world’s electricity before 2030 – and demand for all three fossil fuels – coal, oil and gas – is still projected to peak by the end of the decade. Clean energy is entering the energy system at an unprecedented rate, but deployment is far from uniform across technologies and markets.
In this context, the WEO-2024 also shows that the contours of a new, more electrified energy system are coming into focus as global electricity demand soars. Electricity use has grown at twice the pace of overall energy demand over the last decade, with two-thirds of the global increase in electricity demand over the last ten years coming from China.
“In previous World Energy Outlooks, the IEA made it clear that the future of the global energy system is electric – and now it is visible to everyone,” said Dr Birol. “In energy history, we’ve witnessed the Age of Coal and the Age of Oil – and we’re now moving at speed into the Age of Electricity, which will define the global energy system going forward and increasingly be based on clean sources of electricity.”
“As with many other global energy trends today, China is a major part of what is happening,” Dr Birol added. “Whether it’s investment, fossil fuel demand, electricity consumption, deployment of renewables, the market for EVs, or clean technology manufacturing, we are now in a world where almost every energy story is essentially a China story. Just one example: China’s solar expansion is now proceeding at such a rate that, by the early 2030s – less than ten years from now – China’s solar power generation alone could exceed the total electricity demand of the United States today.”
Global electricity demand growth is set to accelerate further in the years ahead, adding the equivalent of Japanese demand to global electricity use each year in a scenario based on today’s policy settings – and rising even more quickly in scenarios that meet national and global goals for achieving net zero emissions.
For clean energy to continue growing at pace, much greater investment in new energy systems, especially in electricity grids and energy storage, are necessary. Today, for every dollar spent on renewable power, 60 cents are spent on grids and storage, highlighting how essential supporting infrastructure is not keeping pace with clean energy transitions. Secure decarbonisation of the electricity sector requires investment in grids and storage to increase even more quickly than clean generation, and the investment ratio to rebalance to 1:1. Many power systems are currently vulnerable to an increase in extreme weather events, putting a premium on efforts to bolster their resilience and digital security.
Despite growing momentum behind clean energy transitions, the world is still a long way from a trajectory aligned with its net zero goals. Decisions by governments, investors and consumers too often entrench the flaws in today’s energy system, rather than pushing it towards a cleaner and safer path, the report finds. Reflecting the uncertainties in the current energy world, the WEO-2024 includes sensitivity analysis for the speed at which renewables and electric mobility might grow, how fast demand for LNG might rise, and how heatwaves, efficiency policies and the rise of artificial intelligence (AI) might affect electricity demand going forward.
Based on today’s policy settings, global carbon dioxide emissions are set to peak imminently, but the absence of a sharp decline after that means the world is on course for a rise of 2.4 °C in global average temperatures by the end of the century, well above the Paris Agreement goal of limiting global warming to 1.5 °C. The report underlines the inextricable links between risks of energy security and climate change. In many areas of the world, extreme weather events, intensified by decades of high emissions, are already posing profound challenges for the secure and reliable operation of energy systems, including increasingly severe heatwaves, droughts, floods and storms.
A new energy system needs to be built to last, the WEO-2024 emphasises, one that prioritises security, resilience and flexibility, and ensures that benefits of the new energy economy are shared and inclusive. In some regions of the world, high financing costs and project risks are limiting the spread of cost-competitive clean energy technologies to where they are needed most. This is especially the case in developing economies where these technologies can deliver the biggest returns for sustainable development and emissions reductions. Lack of access to energy remains the most fundamental inequity in today’s energy system, with 750 million people – predominantly in sub-Saharan Africa – without access to electricity and over 2 billion without clean cooking fuels.
To address the evolving energy challenges faced by countries around the world, the IEA is convening an International Summit on the Future of Energy Security in the second quarter of 2025. Hosted by the UK government in London, the Summit will assess the existing and emerging risks facing the global energy system, focusing on solutions and opportunities. And to explore the implications of AI for the energy sector, the IEA will host a Global Conference on Energy & AI at its headquarters in Paris on 4 and 5 December. High-level participants will discuss how pioneering AI technologies can change the way the world produces, consumes and distributes energy.
World Energy Outlook 2024 – Explore report
SourceIEA
EMR Analysis
More information on IEA (International Energy Agency): https://www.iea.org + The IEA is at the heart of global dialogue on energy, providing authoritative analysis, data, policy recommendations, and real-world solutions to help countries provide secure and sustainable energy for all.
The IEA was created in 1974 to help co-ordinate a collective response to major disruptions in the supply of oil. While oil security this remains a key aspect of our work, the IEA has evolved and expanded significantly since its foundation.
Taking an all-fuels, all-technology approach, the IEA recommends policies that enhance the reliability, affordability and sustainability of energy. It examines the full spectrum issues including renewables, oil, gas and coal supply and demand, energy efficiency, clean energy technologies, electricity systems and markets, access to energy, demand-side management, and much more.
Since 2015, the IEA has opened its doors to major emerging countries to expand its global impact, and deepen cooperation in energy security, data and statistics, energy policy analysis, energy efficiency, and the growing use of clean energy technologies.
More information on Dr. Fatih Birol (Executive Director, International Energy Agency): https://www.iea.org/contributors/dr-fatih-birol + https://www.linkedin.com/in/fatih-birol/
More information on International Energy Agency Global Conference on Energy & AI (04& 05 December, 2024 – Paris, France): https://www.iea.org/events/global-conference-on-energy-and-ai + This Conference will feed into the IEA’s forthcoming Special Report on Energy and AI and will help to support various political fora where the AI and energy nexus is discussed.
More information on Net Zero by 2050 by the United Nations: https://www.un.org/en/climatechange/net-zero-coalition + Put simply, net zero means cutting greenhouse gas emissions to as close to zero as possible, with any remaining emissions re-absorbed from the atmosphere, by oceans and forests for instance.
Currently, the Earth is already about 1.1°C warmer than it was in the late 1800s, and emissions continue to rise. To keep global warming to no more than 1.5°C – as called for in the Paris Agreement – emissions need to be reduced by 45% by 2030 and reach net zero by 2050.
More than 140 countries, including the biggest polluters – China, the United States, India and the European Union – have set a net-zero target, covering about 88% of global emissions. More than 9,000 companies, over 1000 cities, more than 1000 educational institutions, and over 600 financial institutions have joined the Race to Zero, pledging to take rigorous, immediate action to halve global emissions by 2030.
More information on Net Zero by 2050 by the Science Based Targets initiative (SBTi): https://sciencebasedtargets.org/net-zero + The SBTi’s Corporate Net-Zero Standard is the world’s only framework for corporate net-zero target setting in line with climate science. It includes the guidance, criteria, and recommendations companies need to set science-based net-zero targets consistent with limiting global temperature rise to 1.5°C.
UN vs. SBTi:
- UN targets nations, while SBTi focuses on companies. UN sets a broad goal, while SBTI provides a detailed framework for target setting.
- Both aim to achieve net zero emissions and limit warming to 1.5°C. The UN sets the overall direction, and SBTi helps businesses translate that goal into actionable plans.
Key components of the Corporate Net-Zero Standard:
- Near-term targets: Rapid, deep cuts to direct and indirect value-chain emissions must be the overarching priority for companies. Companies must set near-term science-based targets to roughly halve emission before 2030. This is the most effective, scientifically-sound way of limiting global temperature rise to 1.5°C.
- Long-term targets: Companies must set long-term science-based targets. Companies must cut all possible – usually more than 90% – of emissions before 2050.
- Neutralize residual emissions: After a company has achieved its long-term target and cut emissions by more than 90%, it must use permanent carbon removal and storage to counterbalance the final 10% or more of residual emissions that cannot be eliminated. A company is only considered to have reached net-zero when it has achieved its long-term science-based target and neutralized any residual emissions.
- Beyond Value Chain Mitigation (BVCM): Businesses should invest now in actions to reduce and remove emissions outside of their value chains in addition to near- and long-term science-based targets.
EMR Additional Notes:
- Liquefied Natural Gas (“LNG”):
- LNG fuel, or liquefied natural gas, is a natural gas converted to liquid form through liquefaction. During this process, natural gas is cooled at low temperatures until it turns into a liquid, and the volume of gas is reduced by approximately 600 times.
- When LNG is returned to its gaseous state, it is used across the residential, commercial and industrial sectors for purposes as diverse as heating, cooking, generating electricity and manufacturing a wide variety of products. LNG is also used as a fuel for heavy-duty and other vehicles.
- Differences between LNG & LPG (Also known as propane or butane):
- Light pressure is used to liquefy LPG, while LNG is liquefied cryogenically, i.e. through exposure to extremely low temperatures
- LPG is stored, shipped and transported in tanks or cylinders, whereas LNG is stored and shipped in purpose built cryogenic tanks. Pipelines are usually used to transport LNG.
- The need for cryogenic storage, coupled with infrastructure requirements such as production plants, dispensing stations and pipeline transport facilities, means LNG is not a viable option in many developing nations.
- Grid, Microgrids, DERs and DERM’s:
- The power grid is a network for delivering electricity to consumers. The power grid includes generator stations, transmission lines and towers, and individual consumer distribution lines.
- The grid constantly balances the supply and demand for the energy that powers everything from industry to household appliances.
- Electric grids perform three major functions: power generation, transmission, and distribution.
- A microgrid is a small-scale power grid that can operate independently or collaboratively with other small power grids. The practice of using microgrids is known as distributed, dispersed, decentralized, district or embedded energy production.
- Smart Grid is any electrical grid + IT at all levels . Micro Grid is a group of interconnected loads and DERs (Distributed energy resources) within a clearly defined electrical and geographical boundaries witch acts as a single controllable entity with respect to the main grid.
- Distributed energy resources (DERs) are small-scale electricity supply (typically in the range of 3 kW to 50 MW) or demand resources that are interconnected to the electric grid. They are power generation resources and are usually located close to load centers, and can be used individually or in aggregate to provide value to the grid.
- Common examples of DERs include rooftop solar PV units, natural gas turbines, microturbines, wind turbines, biomass generators, fuel cells, tri-generation units, battery storage, electric vehicles (EV) and EV chargers, and demand response applications.
- Distributed energy resources management systems (DERMS) are platforms which helps mostly distribution system operators (DSO) manage their grids that are mainly based on distributed energy resources (DER).
- DERMS are used by utilities and other energy companies to aggregate a large energy load for participation in the demand response market. DERMS can be defined in many ways, depending on the use case and underlying energy asset.
- Energy Storage System (ESS):
- An energy storage system, often abbreviated as ESS, is a device or group of devices assembled together, capable of storing energy in order to supply electrical energy at a later time. An energy storage system consists of three main components:
- a power conversion system, which transforms electrical energy into another form of energy and vice versa;
- a storage unit, which stores the converted energy;
- a control system, which manages the energy flow between the converter and the storage unit.
- An energy storage system, often abbreviated as ESS, is a device or group of devices assembled together, capable of storing energy in order to supply electrical energy at a later time. An energy storage system consists of three main components:
- Battery Energy Storage System (BESS):
- A BESS is an energy storage system (ESS) that captures energy from different sources, accumulates this energy, and stores it in rechargeable batteries for later use.
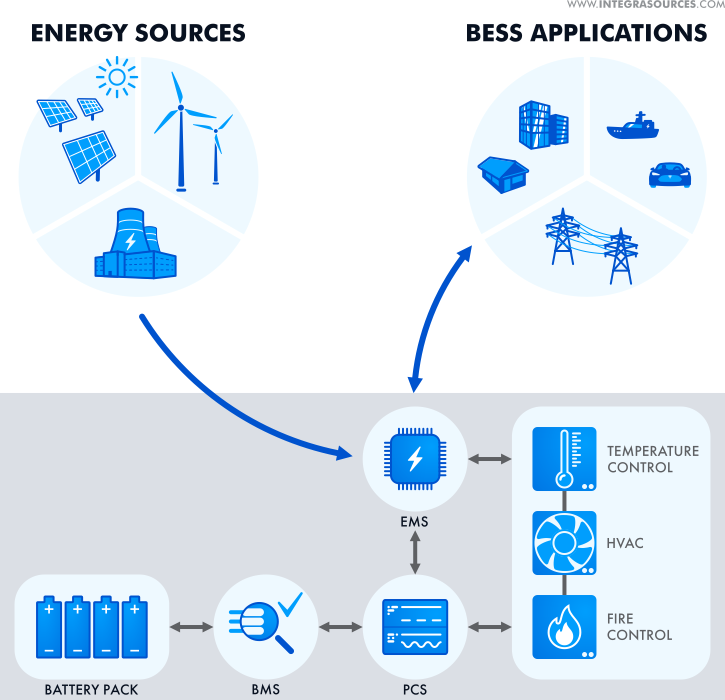
- Carbon Dioxide (CO2):
- Primary greenhouse gas emitted through human activities. Carbon dioxide enters the atmosphere through burning fossil fuels (coal, natural gas, and oil), solid waste, trees and other biological materials, and also as a result of certain chemical reactions (e.g., manufacture of cement). Carbon dioxide is removed from the atmosphere (or “sequestered”) when it is absorbed by plants as part of the biological carbon cycle.
- Biogenic Carbon Dioxide (CO2):
- Biogenic Carbon Dioxide (CO2) and Carbon Dioxide (CO2) are the same. Scientists differentiate between biogenic carbon (that which is absorbed, stored and emitted by organic matter like soil, trees, plants and grasses) and non-biogenic carbon (that found in all other sources, most notably in fossil fuels like oil, coal and gas).
- Decarbonization:
- Reduction of carbon dioxide emissions through the use of low carbon power sources, achieving a lower output of greenhouse gasses into the atmosphere.
- Carbon Footprint:
- There is no universally agreed definition of what a carbon footprint is.
- A carbon footprint is generally understood to be the total amount of greenhouse gas (GHG) emissions that are directly or indirectly caused by an individual, organization, product, or service. These emissions are typically measured in tonnes of carbon dioxide equivalent (CO2e).
- In 2009, the Greenhouse Gas Protocol (GHG Protocol) published a standard for calculating and reporting corporate carbon footprints. This standard is widely accepted by businesses and other organizations around the world. The GHG Protocol defines a carbon footprint as “the total set of greenhouse gas emissions caused by an organization, directly and indirectly, through its own operations and the value chain.”
- CO2e (Carbon Dioxide Equivalent):
- CO2e means “carbon dioxide equivalent”. In layman’s terms, CO2e is a measurement of the total greenhouse gases emitted, expressed in terms of the equivalent measurement of carbon dioxide. On the other hand, CO2 only measures carbon emissions and does not account for any other greenhouse gases.
- A carbon dioxide equivalent or CO2 equivalent, abbreviated as CO2-eq is a metric measure used to compare the emissions from various greenhouse gases on the basis of their global-warming potential (GWP), by converting amounts of other gases to the equivalent amount of carbon dioxide with the same global warming potential.
- Carbon dioxide equivalents are commonly expressed as million metric tonnes of carbon dioxide equivalents, abbreviated as MMTCDE.
- The carbon dioxide equivalent for a gas is derived by multiplying the tonnes of the gas by the associated GWP: MMTCDE = (million metric tonnes of a gas) * (GWP of the gas).
- For example, the GWP for methane is 25 and for nitrous oxide 298. This means that emissions of 1 million metric tonnes of methane and nitrous oxide respectively is equivalent to emissions of 25 and 298 million metric tonnes of carbon dioxide.
- Carbon Capture and Storage (CCS) – Carbon Capture, Utilisation and Storage (CCUS):
- CCS involves the capture of carbon dioxide (CO2) emissions from industrial processes. This carbon is then transported from where it was produced, via ship or in a pipeline, and stored deep underground in geological formations.
- CCS projects typically target 90 percent efficiency, meaning that 90 percent of the carbon dioxide from the power plant will be captured and stored.
- Carbon Dioxide Removal (CDR):
- Carbon Dioxide Removal encompasses approaches and methods for removing CO2 from the atmosphere and then storing it permanently in underground geological formations, in biomass, oceanic reservoirs or long-lived products in order to achieve negative emissions.
- Direct Air Capture (DAC):
- Technologies extracting CO2 directly from the atmosphere at any location, unlike carbon capture which is generally carried out at the point of emissions, such as a steel plant.
- Constraints like costs and energy requirements as well as the potential for pollution make DAC a less desirable option for CO2 reduction. Its larger land footprint when compared to other mitigation strategies like carbon capture and storage systems (CCS) also put it at a disadvantage.
- Carbon Credits or Carbon Offsets:
- Permits that allow the owner to emit a certain amount of carbon dioxide or other greenhouse gases. One credit permits the emission of one ton of carbon dioxide or the equivalent in other greenhouse gases.
- The carbon credit is half of a so-called cap-and-trade program. Companies that pollute are awarded credits that allow them to continue to pollute up to a certain limit, which is reduced periodically. Meanwhile, the company may sell any unneeded credits to another company that needs them. Private companies are thus doubly incentivized to reduce greenhouse emissions. First, they must spend money on extra credits if their emissions exceed the cap. Second, they can make money by reducing their emissions and selling their excess allowances.
- AI – Artificial Intelligence:
- https://searchenterpriseai.techtarget.com/definition/AI-Artificial-Intelligence +
- Artificial intelligence is the simulation of human intelligence processes by machines, especially computer systems.
- As the hype around AI has accelerated, vendors have been scrambling to promote how their products and services use AI. Often what they refer to as AI is simply one component of AI, such as machine learning. AI requires a foundation of specialized hardware and software for writing and training machine learning algorithms. No one programming language is synonymous with AI, but well a few, including Python, R and Java, are popular.
- In general, AI systems work by ingesting large amounts of labeled training data, analyzing the data for correlations and patterns, and using these patterns to make predictions about future states. In this way, a chatbot that is fed examples of text chats can learn to produce lifelike exchanges with people, or an image recognition tool can learn to identify and describe objects in images by reviewing millions of examples.
- AI programming focuses on three cognitive skills: learning, reasoning and self-correction.
- What are the 4 types of artificial intelligence?
- Type 1: Reactive machines. These AI systems have no memory and are task specific. An example is Deep Blue, the IBM chess program that beat Garry Kasparov in the 1990s. Deep Blue can identify pieces on the chessboard and make predictions, but because it has no memory, it cannot use past experiences to inform future ones.
- Type 2: Limited memory. These AI systems have memory, so they can use past experiences to inform future decisions. Some of the decision-making functions in self-driving cars are designed this way.
- Type 3: Theory of mind. Theory of mind is a psychology term. When applied to AI, it means that the system would have the social intelligence to understand emotions. This type of AI will be able to infer human intentions and predict behavior, a necessary skill for AI systems to become integral members of human teams.
- Type 4: Self-awareness. In this category, AI systems have a sense of self, which gives them consciousness. Machines with self-awareness understand their own current state. This type of AI does not yet exist.
- Machine Learning (ML):
- Developed to mimic human intelligence, it lets the machines learn independently by ingesting vast amounts of data, statistics formulas and detecting patterns.
- ML allows software applications to become more accurate at predicting outcomes without being explicitly programmed to do so.
- ML algorithms use historical data as input to predict new output values.
- Recommendation engines are a common use case for ML. Other uses include fraud detection, spam filtering, business process automation (BPA) and predictive maintenance.
- Classical ML is often categorized by how an algorithm learns to become more accurate in its predictions. There are four basic approaches: supervised learning, unsupervised learning, semi-supervised learning and reinforcement learning.
- Deep Learning (DL):
- Subset of machine learning, Deep Learning enabled much smarter results than were originally possible with ML. Face recognition is a good example.
- DL makes use of layers of information processing, each gradually learning more and more complex representations of data. The early layers may learn about colors, the next ones about shapes, the following about combinations of those shapes, and finally actual objects. DL demonstrated a breakthrough in object recognition.
- DL is currently the most sophisticated AI architecture we have developed.
- Computer Vision (CV):
- Computer vision is a field of artificial intelligence that enables computers and systems to derive meaningful information from digital images, videos and other visual inputs — and take actions or make recommendations based on that information.
- The most well-known case of this today is Google’s Translate, which can take an image of anything — from menus to signboards — and convert it into text that the program then translates into the user’s native language.
- Machine Vision (MV):
- Machine Vision is the ability of a computer to see; it employs one or more video cameras, analog-to-digital conversion and digital signal processing. The resulting data goes to a computer or robot controller. Machine Vision is similar in complexity to Voice Recognition.
- MV uses the latest AI technologies to give industrial equipment the ability to see and analyze tasks in smart manufacturing, quality control, and worker safety.
- Computer Vision systems can gain valuable information from images, videos, and other visuals, whereas Machine Vision systems rely on the image captured by the system’s camera. Another difference is that Computer Vision systems are commonly used to extract and use as much data as possible about an object.
- Generative AI (GenAI):
- Generative AI technology generates outputs based on some kind of input – often a prompt supplied by a person. Some GenAI tools work in one medium, such as turning text inputs into text outputs, for example. With the public release of ChatGPT in late November 2022, the world at large was introduced to an AI app capable of creating text that sounded more authentic and less artificial than any previous generation of computer-crafted text.
- https://searchenterpriseai.techtarget.com/definition/AI-Artificial-Intelligence +
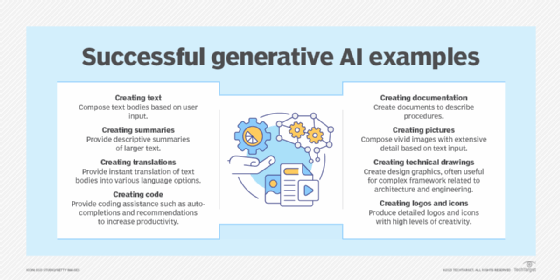
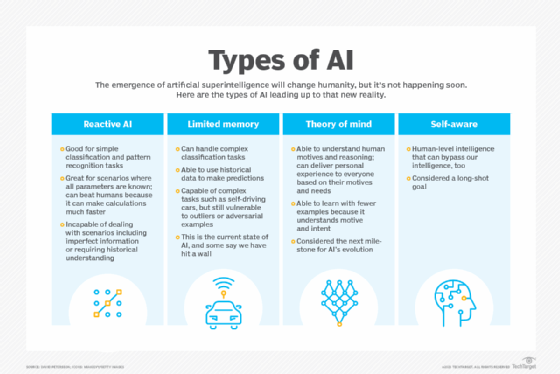
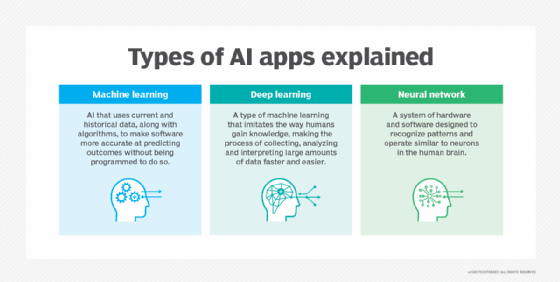
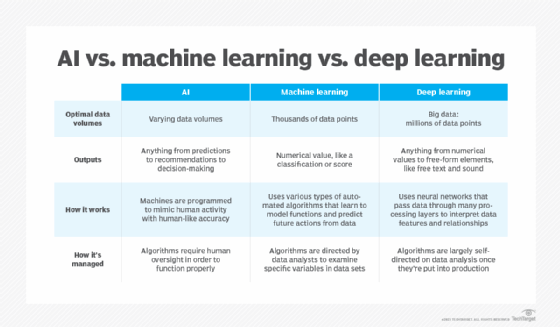
- Edge AI Technology:
- Edge artificial intelligence refers to the deployment of AI algorithms and AI models directly on local edge devices such as sensors or Internet of Things (IoT) devices, which enables real-time data processing and analysis without constant reliance on cloud infrastructure.
- Simply stated, edge AI, or “AI on the edge“, refers to the combination of edge computing and artificial intelligence to execute machine learning tasks directly on interconnected edge devices. Edge computing allows for data to be stored close to the device location, and AI algorithms enable the data to be processed right on the network edge, with or without an internet connection. This facilitates the processing of data within milliseconds, providing real-time feedback.
- Self-driving cars, wearable devices, security cameras, and smart home appliances are among the technologies that leverage edge AI capabilities to promptly deliver users with real-time information when it is most essential.
- Multimodal Intelligence and Agents:
- Subset of artificial intelligence that integrates information from various modalities, such as text, images, audio, and video, to build more accurate and comprehensive AI models.
- Multimodal capabilities allows to interact with users in a more natural and intuitive way. It can see, hear and speak, which means that users can provide input and receive responses in a variety of ways.
- An AI agent is a computational entity designed to act independently. It performs specific tasks autonomously by making decisions based on its environment, inputs, and a predefined goal. What separates an AI agent from an AI model is the ability to act. There are many different kinds of agents such as reactive agents and proactive agents. Agents can also act in fixed and dynamic environments. Additionally, more sophisticated applications of agents involve utilizing agents to handle data in various formats, known as multimodal agents and deploying multiple agents to tackle complex problems.
- The Paris Agreement (COP 21):
- The Paris Agreement is a legally binding international treaty on climate change under UNFCC. It was adopted by 196 Parties at COP 21 in Paris, on 12 December 2015 and entered into force on 4 November 2016.
- Its goal is to limit global warming to well below 2, preferably to 1.5 degrees Celsius, compared to pre-industrial levels.
- To achieve this long-term temperature goal, countries aim to reach global peaking of greenhouse gas emissions as soon as possible to achieve a climate neutral world by mid-century.
- The Paris Agreement is a landmark in the multilateral climate change process because, for the first time, a binding agreement brings all nations into a common cause to undertake ambitious efforts to combat climate change and adapt to its effects.
- The Paris Agreement is a legally binding international treaty on climate change under UNFCC. It was adopted by 196 Parties at COP 21 in Paris, on 12 December 2015 and entered into force on 4 November 2016.